In the realm of energy storage, Lead Carbon Batteries have emerged as a noteworthy contender, finding significant applications in sectors such as renewable energy storage and backup power systems. Their unique composition offers a blend of the traditional lead-acid battery’s robustness with the supercapacitor’s cycling capabilities. This unique feature set, however, demands specific considerations when it comes to storage.
Table 1.1: Overview of Battery Types and Their Characteristics
Battery Type | Average Lifespan | Common Uses | Special Storage Considerations |
---|---|---|---|
Lead Carbon | 8-10 years | Backup power, renewable energy storage | Temperature-sensitive, Avoid prolonged full discharge |
Lithium-ion | 10-15 years | Electronics, Electric vehicles | Avoid extreme temperatures, Protect from puncture |
Nickel-metal hydride | 5-7 years | Electronics, Hybrid vehicles | Store in a cool, dry place |
Alkaline | 5-8 years | Household devices | Store in a cool, dry place, Avoid exposure to moisture |
This comprehensive guide is tailored to demystify Lead Carbon Batteries, providing insights into their functioning, advantages, and best practices for storage. As renewable energy solutions become increasingly prevalent, understanding the nuances of storing these batteries effectively is more critical than ever. Through subsequent chapters, we shall navigate the intricacies of these batteries, ensuring that readers gain a holistic perspective on their optimal storage and usage.
Contents
- 1 What is a Lead Carbon Battery?
- 2 The Science Behind Lead Carbon Batteries
- 3 Benefits of Using Lead Carbon Batteries
- 4 Key Considerations for Storing Lead Carbon Batteries
- 5 Comparative Analysis: Lead Carbon Batteries vs. Other Battery Types
- 6 Practical Applications of Lead Carbon Batteries
- 7 Lead Carbon Battery Maintenance and Safety
- 8 Conclusion: Summing Up the World of Lead Carbon Batteries
What is a Lead Carbon Battery?
Lead Carbon Batteries (LCB) are a relatively recent development in the world of energy storage. They combine the traits of traditional lead-acid batteries with those of carbon-based supercapacitors. But what sets them apart from other batteries, and why are they garnering attention?
Table 2.1: Components of Lead Carbon Battery
Component | Role | Material Used |
---|---|---|
Positive Plate | Holds the charge, undergoes chemical reactions during charging | Lead dioxide (PbO2) |
Negative Plate | Discharges the stored energy | Carbon and Lead (Pb) |
Electrolyte | Facilitates the movement of ions, enabling the battery to function | Diluted sulfuric acid (H2SO4) |
Separator | Separates the positive and negative plates to prevent short circuits | Micro-porous material |
The unique blend of lead and carbon in the negative plate is the defining feature of these batteries. This combination offers several advantages over traditional lead-acid batteries:
- Enhanced Cycle Life: Due to the inclusion of carbon, LCBs demonstrate a longer cycle life, making them more cost-effective in applications that require frequent charging and discharging.
- Superior Partial State of Charge (PSoC) Performance: One of the Achilles heels of traditional lead-acid batteries is their reduced longevity under partial state of charge conditions. LCBs, with their carbon inclusion, can handle PSoC scenarios more efficiently.
- Rapid Charge Capability: The carbon component improves the charge acceptance of the battery. This means that Lead Carbon Batteries can be charged faster than their traditional counterparts.
- Decreased Sulfation: Sulfation is the formation of lead sulfate crystals on the battery plates, which is a common issue in lead-acid batteries. The carbon in LCBs significantly reduces this problem, enhancing the battery’s lifespan.
Table 2.2: Lead Carbon Battery vs. Traditional Lead-Acid Battery
Feature | Lead Carbon Battery | Traditional Lead-Acid Battery |
---|---|---|
Cycle Life | Longer | Shorter |
PSoC Performance | Superior | Inferior |
Charge Speed | Faster | Slower |
Sulfation Risk | Lower | Higher |
In summary, while Lead Carbon Batteries build upon the foundational principles of lead-acid batteries, they introduce carbon into the equation, yielding a product with enhanced performance and longevity. This makes them particularly appealing for scenarios requiring durable and dependable energy storage.
As we delve deeper into the science behind these batteries in the next chapter, we’ll gain a clearer understanding of their workings and the unique attributes that make them stand out in the crowded world of energy storage solutions.

The Science Behind Lead Carbon Batteries
The science of a Lead Carbon Battery, like any battery, revolves around electrochemical reactions. However, the introduction of carbon into the equation brings a new dimension to these reactions. Let’s unpack the fundamental processes.
3.1 Electrochemical Reactions
Every battery operates through a series of chemical reactions that allow for the storage and release of energy. In a Lead Carbon Battery:
- Charging Phase: The battery converts electrical energy into chemical energy.
- Positive Plate Reaction: PbO2+3H2SO4→PbSO4+2H2O+O2
- Negative Plate Reaction: Pb+H2SO4→PbSO4+H2
- Discharging Phase: The stored chemical energy is converted back into electrical energy to power devices.
- Positive Plate Reaction: PbSO4+2H2O→PbO2+3H2SO4+O2
- Negative Plate Reaction: PbSO4+H2→Pb+H2SO4
3.2 Role of Carbon
Carbon’s addition modifies the electrochemical dynamics of the battery:
- Double-layer Capacitance: The carbon, acting similar to a supercapacitor, stores charges at the boundary between the electrolyte and the carbon surface. This double-layer capacitance contributes to the battery’s rapid charge capability.
- Improved Conductivity: Carbon enhances the conductivity of the battery plates, allowing for faster electron movement. This translates to better overall battery performance.
- Reduced Sulfation: Carbon’s inclusion mitigates the risk of sulfation. As the battery operates, lead sulfate can accumulate on the plates. Carbon aids in preventing this accumulation, thereby extending battery life.
Table 3.1: Unique Properties of Lead Carbon Battery Induced by Carbon
Property/Feature | Role of Carbon |
---|---|
Double-layer Capacitance | Offers rapid charge capability |
Enhanced Conductivity | Improves overall battery performance |
Sulfation Resistance | Reduces the accumulation of lead sulfate, extending life |
3.3 Synergy between Lead and Carbon
While both lead and carbon have their individual strengths, their combination in a Lead Carbon Battery offers a synergy that neither could achieve on its own. Lead provides the robust, time-tested energy storage capability, while carbon lends its rapid charging and discharging attributes. Together, they create a battery that is both durable and efficient.
Benefits of Using Lead Carbon Batteries
As energy storage becomes an integral part of modern infrastructure, especially with the surge in renewable energy adoption, the need for reliable and efficient batteries has never been greater. Lead Carbon Batteries (LCBs), emerging as a frontrunner in this race, present a myriad of advantages over traditional battery types.
4.1 Extended Life Cycle
LCBs consistently showcase a longer life cycle compared to traditional lead-acid batteries. This is primarily due to the carbon’s inclusion, which reduces the negative impacts of frequent charge-discharge cycles on the battery’s health.
Table 4.1: Estimated Life Cycles of Battery Types
Battery Type | Estimated Cycle Life at 50% Depth of Discharge |
---|---|
Lead Carbon | 1500 – 2000 cycles |
Traditional Lead-Acid | 300 – 500 cycles |
Lithium-ion | 1000 – 1500 cycles |
Nickel-metal hydride | 500 – 800 cycles |
4.2 Superior Partial State of Charge (PSoC) Tolerance
A common downfall of many battery types is their susceptibility to degradation when frequently operated in a partial state of charge. LCBs, however, excel in this domain, displaying minimal wear even with regular PSoC use. This makes them ideal for solar applications, where batteries might not always achieve full charge during cloudy or rainy days.
4.3 Enhanced Charge Acceptance
The carbon component of LCBs promotes faster electron movement, translating to rapid charge capabilities. This means that these batteries can be replenished more quickly than their non-carbon counterparts, a trait particularly valuable in scenarios demanding quick turnaround times, such as backup power systems.
4.4 Reduced Sulfation Risk
Sulfation, the formation of lead sulfate crystals, is a primary cause of failure in lead-acid batteries. The carbon in LCBs significantly reduces sulfation, ensuring longevity and consistent performance.
4.5 Environmental Resilience
While no battery can be deemed entirely ‘eco-friendly’, LCBs do present a lesser environmental burden compared to some alternatives. The combination of a longer lifecycle and reduced sulfation means fewer batteries end up as waste, alleviating the environmental impact.
Table 4.2: Environmental Impact of Batteries
Feature | Lead Carbon Battery | Traditional Lead-Acid Battery | Lithium-ion |
---|---|---|---|
Lifespan | Longer | Shorter | Moderate |
Toxic Components | Moderate | High | Moderate |
Recyclability | High | High | Moderate |
In conclusion, Lead Carbon Batteries offer an amalgamation of traditional robustness with modern efficiencies. Their advantages, spanning from extended lifecycles to environmental resilience, position them as a compelling choice for a range of applications, from renewable energy storage to uninterruptible power supplies.
Key Considerations for Storing Lead Carbon Batteries
Storing Lead Carbon Batteries (LCBs) properly is paramount to harnessing their full potential and ensuring their longevity. By adhering to the best storage practices, one can avoid common pitfalls that might degrade the battery or shorten its lifespan. Here, we explore the primary considerations one should be mindful of when storing LCBs.
5.1 Temperature Control
LCBs, like most batteries, are sensitive to extreme temperatures. Ensuring a stable, moderate temperature is essential for prolonging their operational life.
- Optimal Storage Temperature: It’s best to store LCBs in a range of 20°C to 25°C (68°F to 77°F).
- Avoid Extremes: Temperatures below freezing can cause the electrolyte to freeze, resulting in potential damage. Similarly, high temperatures can accelerate the battery’s self-discharge rate and may lead to a shortened lifespan.
Table 5.1: Temperature Effects on LCBs
Temperature Range | Impact on LCBs |
---|---|
Below 0°C | Risk of electrolyte freezing; potential damage |
0°C – 20°C | Slower self-discharge; prolonged storage possible |
20°C – 25°C | Ideal storage range |
Above 25°C | Accelerated self-discharge; potential for overheating |
5.2 Charge State
It’s advisable to store LCBs at a partial charge rather than a full charge or complete discharge.
- Recommended State: A 50-60% charge is ideal for long-term storage.
- Avoid Full Discharge: Storing the battery in a fully discharged state can lead to sulfation, impacting its performance and lifespan.
5.3 Physical Placement
Proper positioning and handling of the battery can prevent accidental damages:
- Upright Position: Always store LCBs in an upright position to prevent leakage.
- Avoid Stacking: If you must stack batteries, ensure it’s done safely to avoid any potential for crushing or damaging the casings.
- Separation: Maintain some distance between individual batteries to facilitate air circulation and prevent overheating.
5.4 Humidity and Environmental Factors
LCBs should be kept in a dry environment.
- Ideal Humidity: Aim for a relative humidity of less than 60%.
- Avoid Moisture: Exposure to excessive moisture or direct water can damage the battery and its terminals.
Table 5.2: Environmental Considerations for LCBs
Environmental Factor | Ideal Condition | Impact of Neglect |
---|---|---|
Humidity | Below 60% relative humidity | Corrosion of terminals, reduced performance |
Moisture | Dry environment | Damage to battery internals, short circuits |
Comparative Analysis: Lead Carbon Batteries vs. Other Battery Types
In the world of energy storage, there’s no one-size-fits-all solution. Various battery types cater to different needs, each coming with its unique set of advantages and limitations. To truly appreciate the value proposition of Lead Carbon Batteries (LCBs), we need to juxtapose them with other prevalent battery technologies.
6.1 Lead Carbon Batteries vs. Traditional Lead-Acid Batteries
Table 6.1: Comparative Traits of LCBs and Traditional Lead-Acid Batteries
Feature | Lead Carbon Battery | Traditional Lead-Acid Battery |
---|---|---|
Life Cycle | 1500-2000 cycles | 300-500 cycles |
Charge Rate | Faster due to carbon | Slower |
Sulfation Risk | Reduced | High |
PSoC Tolerance | Superior | Moderate |
Cost | Slightly higher | Lower |
Energy Density | Comparable | Comparable |
The primary advantage of LCBs over traditional lead-acid batteries lies in their enhanced life cycle and reduced sulfation risk, attributed to the addition of carbon.
6.2 Lead Carbon Batteries vs. Lithium-ion Batteries
Table 6.2: Comparative Traits of LCBs and Lithium-ion Batteries
Feature | Lead Carbon Battery | Lithium-ion Battery |
---|---|---|
Life Cycle | 1500-2000 cycles | 1000-1500 cycles |
Charge Rate | Fast | Very Fast |
Safety Concerns | Moderate | Concerns with overheating |
Cost | Moderate | Generally higher |
Energy Density | Lower | High |
Recyclability | High | Moderate |
While Lithium-ion batteries boast a higher energy density and rapid charging capabilities, LCBs tend to be more recyclable and offer a competitive life cycle at a potentially lower cost.
6.3 Lead Carbon Batteries vs. Nickel-metal Hydride (NiMH) Batteries
Table 6.3: Comparative Traits of LCBs and NiMH Batteries
Feature | Lead Carbon Battery | NiMH Battery |
---|---|---|
Life Cycle | 1500-2000 cycles | 500-800 cycles |
Charge Rate | Fast | Moderate |
Environmental Impact | Moderate | Lower (fewer toxic components) |
Cost | Moderate | Comparable |
Energy Density | Comparable | Slightly lower |
While NiMH batteries are praised for their lower environmental footprint, LCBs offer a significantly longer life cycle and faster charge rates.
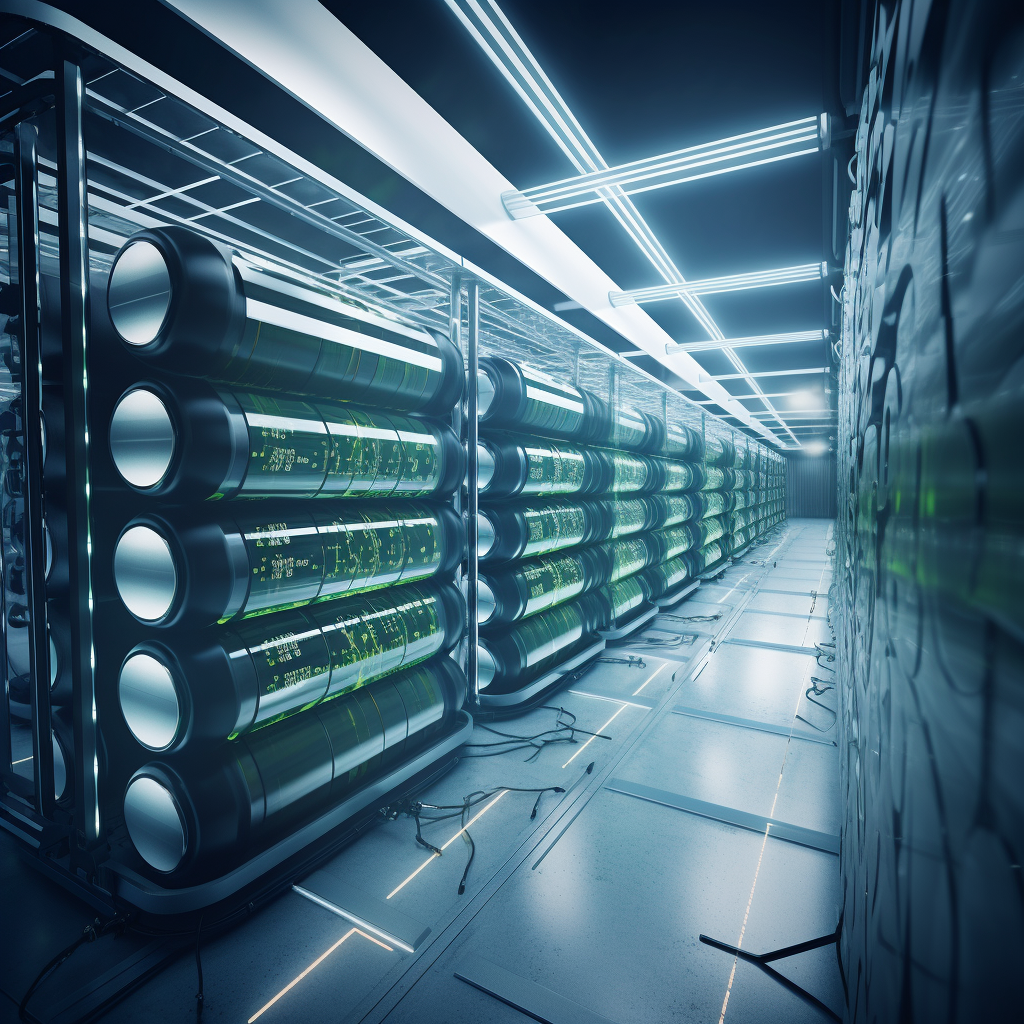
Practical Applications of Lead Carbon Batteries
As the energy landscape continues to evolve, LCBs have found applications across diverse sectors, driven by their unique blend of features. Let’s uncover the realms where Lead Carbon Batteries truly stand out.
7.1 Renewable Energy Storage
With the push towards greener energy sources, LCBs have become increasingly relevant. Their impressive PSoC tolerance and life cycle make them apt for storing energy generated from solar panels and wind turbines.
7.2 Backup Power Supplies
In settings where uninterrupted power is critical—like hospitals or data centers—LCBs act as reliable backup power sources, thanks to their quick charging capabilities and longevity.
7.3 Off-grid Solutions
For remote locations without access to centralized electricity grids, LCBs serve as a power source, often paired with renewable energy installations.
7.4 Transportation
Though not as common as Lithium-ion, LCBs are still employed in certain electric vehicles (EVs) and hybrid applications, offering a balance between cost and performance.
7.5 Grid Stabilization
By acting as a buffer, LCBs can help stabilize power grids, absorbing excess energy during peak production times and releasing it during high demand.
Lead Carbon Battery Maintenance and Safety
To truly maximize the potential of Lead Carbon Batteries (LCBs), proper maintenance and adherence to safety protocols are indispensable. Below are the crucial guidelines and best practices for the care and safe handling of LCBs.
8.1 Regular Inspection
Routine checks can prevent potential issues from escalating.
- Visual Checks: Regularly inspect the battery for any signs of bulging, leakage, or damage.
- Terminal Checks: Ensure that the terminals are clean and free from corrosion.
8.2 Proper Handling
Safe handling prevents accidents and prolongs battery life.
- Wear Protective Gear: When handling batteries, always wear gloves and safety goggles to prevent direct contact with battery acids or other chemicals.
- Lifting Mechanism: Due to their weight, always use proper lifting techniques or equipment to prevent strain or injury.
8.3 Charging Guidelines
Correct charging is key to maximizing battery life and performance.
- Use Compatible Chargers: Ensure that the charger is suited for LCBs to avoid overcharging or undercharging.
- Monitor Charging: Do not leave the battery unattended during charging. Ensure it doesn’t overheat.
8.4 Storage Safety
If the battery is to be stored for an extended period:
- Store Upright: Always ensure the battery remains in an upright position.
- Keep Away from Combustibles: Store the batteries away from flammable materials to minimize fire risks.
8.5 Emergency Procedures
In the event of battery leakage or other malfunctions:
- Neutralize Spills: For acid spills, use baking soda to neutralize.
- Ventilate Area: If gases are released, ensure good ventilation to dilute harmful fumes.
- Seek Professional Help: In case of severe malfunctions, contact professionals or the battery manufacturer.
Table 8.1: Maintenance and Safety Quick Tips
Aspect | Guideline |
---|---|
Inspection | Conduct monthly visual and terminal checks. |
Handling | Use gloves, goggles, and proper lifting techniques. |
Charging | Use LCB-compatible chargers and monitor the process. |
Storage | Keep upright and away from flammable items. |
Emergencies | Neutralize acid spills and ensure proper ventilation. |
Conclusion: Summing Up the World of Lead Carbon Batteries
As we wind down this comprehensive guide on Lead Carbon Batteries (LCBs), let’s revisit the salient points, encapsulating the essence of this advanced energy storage solution.
9.1 The Unique Blend of LCBs
LCBs stand as a testament to innovative engineering, merging the reliability of lead-acid batteries with the enhanced performance attributes of carbon. This blend furnishes the LCB with superior partial state of charge (PSoC) tolerance, reduced sulfation risk, and a commendable life cycle.
9.2 Storage Essentials
The longevity and efficacy of LCBs are heavily reliant on storage conditions. Keeping them in controlled temperatures, within the optimal charge range, and in dry environments is paramount for harnessing their full potential.
9.3 Comparative Merits
Against the backdrop of various battery technologies, LCBs carve their niche. While they might not match the energy density of lithium-ion batteries or the eco-friendliness of Nickel-metal Hydride (NiMH) batteries, their balanced blend of cost-effectiveness, recyclability, and life cycle makes them a formidable contender.
9.4 Maintenance and Safety
A proactive approach to battery care can vastly amplify the lifespan and performance of LCBs. From regular inspections to adhering to safety protocols during charging and storage, due diligence can prevent potential mishaps and ensure optimal battery health.
Table 9.1: Key Takeaways from the Guide
Highlight | Insight |
---|---|
LCB’s Composition | Fusion of lead and carbon for enhanced performance |
Storage Practices | Control temperature, charge, and humidity |
Comparative Analysis | LCBs’ balanced profile vs. other battery types |
Safety and Maintenance | Routine checks, correct charging, and safe handling |
In closing, Lead Carbon Batteries emerge as a pivotal force in the energy storage arena, bridging traditional attributes with modern enhancements. Their role, especially in renewable energy sectors and off-grid solutions, is set to burgeon in the coming years. As with any technology, understanding its nuances, strengths, and limitations is the key to unlocking its full potential. We hope this guide has equipped you with the knowledge to navigate the world of LCBs effectively.